February 10, 2022 at 12:12 pm | Updated March 16, 2022 at 4:58 pm | 8 min read
What is Biomass Accumulation?
Biomass accumulation is often confused with productivity. Hence it is important to make a distinction between the two processes.
Productivity is the result of photosynthesis. It is measured by photosynthetic rate and by considering the factors that affect it.
Biomass accumulation is the change in biomass over time per unit area. So, to calculate biomass accumulation, we have to consider the processes in the production, use, and distribution of biomass in plants.
Subscribe to the CID Bio-Science Weekly article series.
By submitting this form, you are consenting to receive marketing emails from: . You can revoke your consent to receive emails at any time by using the SafeUnsubscribe® link, found at the bottom of every email. Emails are serviced by Constant Contact
Biomass accumulation can be influenced by
- Plant attributes like morphology, genetics, and physiology.
- Environmental factors like air temperature, humidity, light intensity, and shading.
- Soil conditions such as temperature, soil moisture, nutrient availability, soil type, compaction, etc.
- Management practices in food production, forestry, silviculture, and ecosystem protection are larger-scale factors that would help biomass production.
Researchers measure biomass accumulation on various scales:
- The whole plant (above-ground and below-ground plant parts)
- Field or local scale
- Large ecosystem scale
The last two categories will include the “whole-plant” category while also considering additional large-scale factors–usually management practices, climate, geography, etc.
This article will focus on the processes that change biomass accumulation at the plant level.
Morphological Features
The morphological features that affect biomass accumulation are plant architecture, leaf area, and the root system.
Architecture of Plants
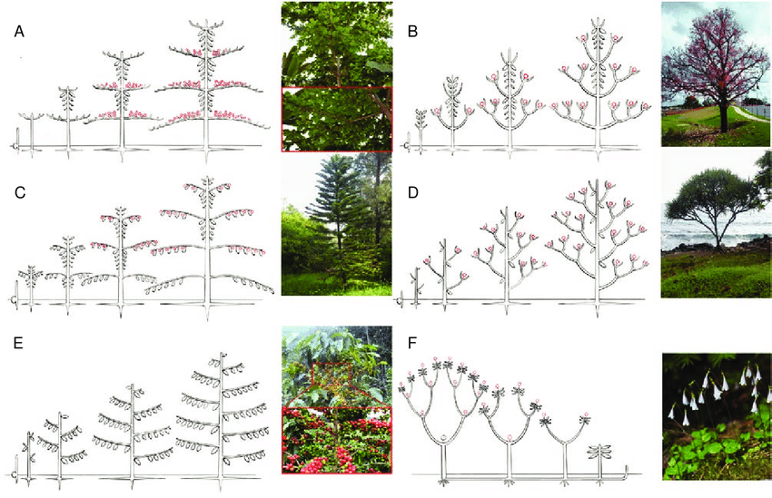
Plant architecture shows the pattern in which plants grow to increase light interception. It is defined as the three-dimensional organization of above-ground plant organs, such as the branching pattern, arrangement, shape, and size of leaves, flowers, and fruits. It was and remains one of the primary methods to understand how they adapt to their environment.
- Growth patterns can be determinate or indeterminate. They can also be horizontal (as in creeping plants) or vertical.
- Branching can be defined based on the site, timing, symmetry, and growth pattern in the plants to produce various shapes, a few of which are shown in Figure 1. Branching patterns also extend to the arrangement of leaves, for example, alternate or opposite leaves, etc.
Plant architecture directly determines the canopy of the entire plant, in turn affecting the plant’s light interception efficiency. Plant canopy imagers can easily measure these structures, giving vital insight into plant processes. Among other essential parameters, these tools can record Leaf Area Index (one-sided leaf area per unit area of land) and photosynthetically active radiation (PAR) that infiltrates through the canopy.
Leaf Area
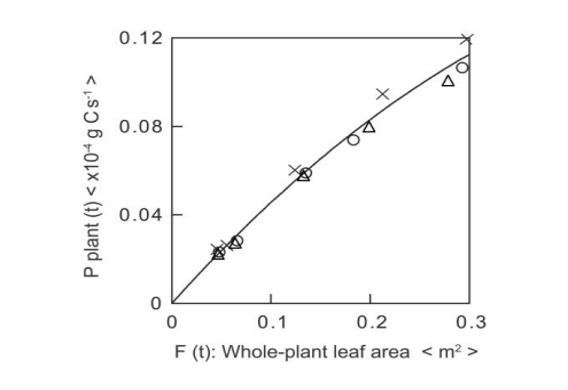
Leaves are the most vital parts of a plant for biomass production, as this is the site where photosynthesis occurs. Besides arrangement and shape, leaf area is crucial in determining the amount of light intercepted by plants for photosynthesis and, therefore, the amount of food produced by the plant. The amount of light intercepted by each leaf will decrease with age due to self-shading and also affect the photosynthetic rate. Thus there are diminishing returns from the leaf area, as shown in Figure 2.
Other gas exchanges like transpiration and stomatal conductance occur in the leaves, and the leaf area is involved in water loss. Hence, plants living in dry environments have reduced or adapted leaves to limit plant water loss to survive. For example, cacti have leaves reduced to spines. The flattened stems have chlorophyll to conduct photosynthesis.
Many abiotic and biotic factors that reduce leaf area will influence productivity, such as drought, lack of soil moisture, high temperatures, or pests and diseases. The location of the plant in an ecosystem and the shading it suffers from can also reduce the contribution of leaf area to biomass production and accumulation.
Agricultural practices such as correct spacing, irrigation, and nutrition attempt to optimize leaf area and crop productivity.
Leaf area and leaf area index are among the most common and widely used parameters in agricultural, ecological, and forest sciences. Portable leaf area meters that can take non-destructive and repeat measurements in the field have further improved the use of these parameters.
Root System

The root system has many functions, like anchoring the plant in the soil, absorbing water and nutrients, storing food, and vegetative propagation. There are various types of roots for these functions.
The adventitious roots, lateral roots, and tubers are relevant for biomass accumulation, see Figure 3. In all these cases, the root system architecture (RSA), which considers root length, number, spread, and length of lateral roots, shows a great degree of plasticity to respond to soil and environmental conditions, like temperature, rainfall, elevation, and geographical location.
Roots, especially fine roots, will also experience seasonal and yearly variations in growth due to water and nutrient availability. This plasticity is crucial in enhancing nutrient and water use efficiency to improve productivity. Agriculturists take advantage of this phenomenon when they choose irrigation methods. It can also help plants in natural ecosystems to adapt to increasing drought due to climate change.
The biomass accumulation in the roots is not static and can differ according to seasons and stress. Usually, plants in drought conditions invest more biomass to grow deeper or wider root systems to improve their access to water. Similarly, in colder regions, where perennial herbs lose their above-ground structures or leaves, nutrients like nitrogen are allotted to the barks of roots and stems.
Researchers can now efficiently study root growth, distribution, and turnover with minirhizotrons in combination with easy-install transparent root tubes.
Roots use half the photosynthates produced by the plants in search of nutrients. So they play a role in biomass production and use in plants.
Physiological Processes
The physiological processes important in determining biomass accumulation are the gas exchanges -photosynthesis, transpiration, stomatal conductance, and respiration. These can be measured by Infra-red Gas Analyzers.
Photosynthesis
Biomass production depends mainly on photosynthesis, as 90% of the plant biomass is made of carbon, hydrogen, and oxygen. So the rate of photosynthesis is important to determine productivity.
Photoassimilates produced at the source (leaves) are sent to other parts of the plants for maintenance and growth, after which the excess is stored. The rate of photosynthesis depends on intrinsic factors such as the chlorophyll content, internal CO2 levels, stomatal conductance, and number, area, and age of leaves, see Figure 4.
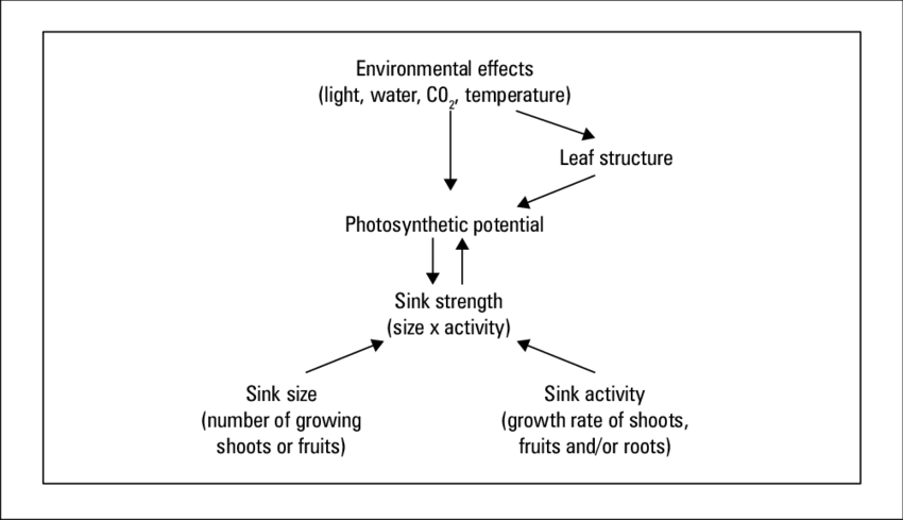
External factors like light availability, temperatures, and soil water will influence the rate of photosynthesis. Low light levels reduce photosynthesis, but high light intensity will damage the photosynthetic apparatus. Very high temperatures can upset enzymatic reactions, and low water causes the stomata to close and reduces CO2 availability for photosynthesis.
Photosynthetic rate can be measured by chlorophyll fluorescence and the exchange of CO2 and O2 that occurs in the process. Usually, the two processes are measured simultaneously to get more information on plant health that could influence biomass accumulation.
Transpiration and Stomatal Conductance
The two other gas exchanges in the leaves, transpiration and stomatal conductance, are intricately connected with carbon assimilation, carbon metabolism, and nutrient absorption.
Transpiration is the loss of excess plant moisture, which occurs through stomata.
Stomata are openings on the dorsal (underside) surface of leaves formed by guard cells. When they are turgid due to higher cell moisture content, they open and allow excess water vapor that has accumulated in the leaf tissue nearby to diffuse out depending on the external temperature and humidity. Conversely, the guard cells become flaccid and close when the cell moisture levels are low due to less water availability.
Since CO2 from the air used in photosynthesis diffuses into the leaves through stomata, transpiration and stomatal conductance influence the rate of photosynthesis and determine plant productivity.
On the other hand, oxygen necessary for carbon metabolism during respiration is also taken in by the plants through stomata.
Transpiration also produces the pressure that absorbs water and dissolved nutrients from the soil through the roots. Fourteen nutrients are derived from the soils, including major nutrients like nitrogen, phosphorus, potassium, and minor nutrients (calcium, magnesium, boron, zinc, manganese, iron, copper, molybdenum, nickel, zinc, sulfur, and chlorine). These are necessary for producing bio compounds like chlorophyll, proteins, enzymes, vitamins, etc., which the plants need for various crucial physiological processes, see Table 1.
These nutrients help produce biomass and are also part of the compounds in the dry matter of plants.
Table 1: Use of the 14 soil-derived nutrients for plant growth and productivity, Mahler. (Credits: https://www.extension.uidaho.edu/publishing/pdf/CIS/CIS1124.pdf)
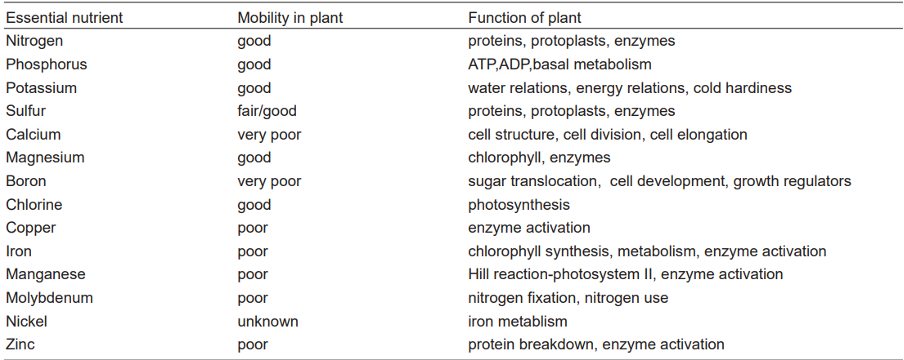
Thus these two gas exchanges are essential for water and nutrient use efficiency and are regulated during crop breeding programs to produce cultivars that achieve an optimum balance between stomatal conductance and transpiration with photosynthesis.
Carbohydrate Metabolism
The carbon assimilated by plants serves as food. Plants use the photosynthates (glucose and starch) to produce energy, grow, maintain their structure, and reproduce.
Plants produce energy through respiration, which uses up to 60% of the food produced by photosynthesis. The process occurs in the mitochondria of cells in all parts of the plants, including roots. Oxygen breaks down the food to release energy and CO2. Some CO2 is used for photosynthesis, but most is released into the atmosphere. Depending on where the energy is used, respiration is classified as:
- Maintenance respiration
- Growth respiration
Part of the photosynthates combine with other minerals and compounds to produce larger bio compounds like proteins, pigments, nutraceuticals, etc.
- These could be used to produce new tissue to grow and add height, new branches, leaves, flowers, etc.
- These photoassimilates are also needed to increase the plant’s width and make the stem broader and steadier each year in trees.
- Part of the food is used to produce flowers for reproduction.
- The remaining photosynthates are sent to fruits, seeds, root tubers and bulbs, and storage tissues in stems that act as sinks.
As Figure 5 shows, the biomass of interest in agriculture is the grains, fruits, or even flowers. For a forester, the biomass accumulated in the entire tree is interesting. Climate scientists are interested in biomass on an ecosystem scale to establish carbon flux and sequestration.
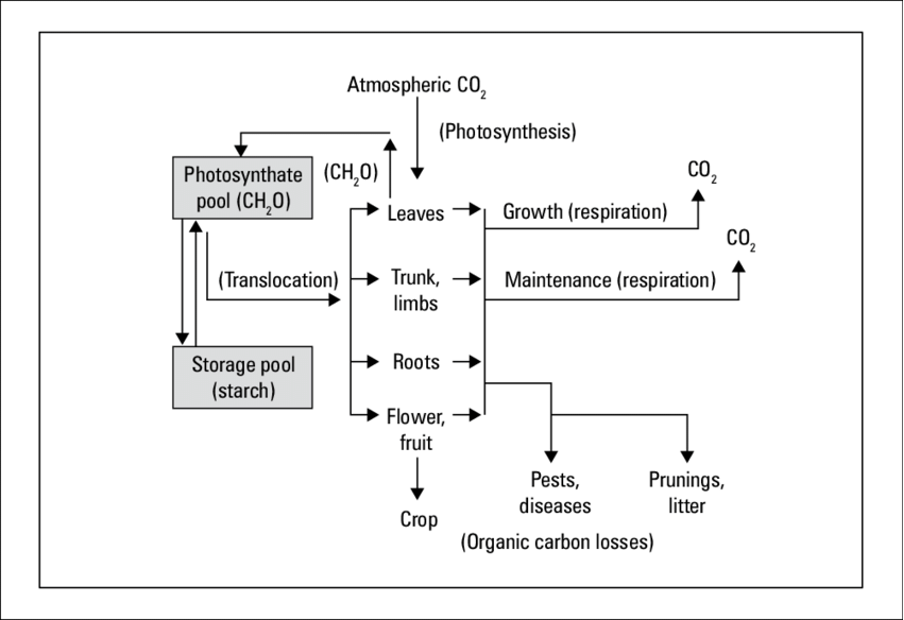
Thus biomass accumulation depends on the production, use, allocation, and storage of photosynthates and other compounds that make up the dry matter.
Genetics
Last but not least, the genotype of plants is crucial. It will determine the characteristics of shoot and root systems, leaf area, type of photosynthesis (C3 or C4 or CAM), and gas exchange rates. Crop scientists use several methods to produce cultivars to improve productivity and cope with stress (biotic and abiotic) that can reduce productivity and ultimately biomass accumulation.
Phenotype plasticity, or the ability of plants to adjust to local environmental conditions by varying morphology and physiology, is valuable, especially for flora in natural systems to adapt to various niches. Phenotype plasticity is also being increasingly explored in crops to produce varieties adapted to global warming and drought.
Management Practices
People strive to provide the right amounts of nutrients and water to ensure that grains, fruits, vegetables, and forest plantations have the ideal conditions to produce and accumulate biomass. In natural ecosystems, management practices focus on protecting forests and other habitats by limiting or eliminating logging, grazing, or clearing to allow the plants to remain standing and accumulate biomass.
—
Vijayalaxmi Kinhal
Science Writer, CID Bio-Science
Ph.D. Ecology and Environmental Science, B.Sc Agriculture
Related Products
Most Popular Articles
- Transpiration in Plants: Its Importance and Applications
- Leaf Area – How & Why Measuring Leaf Area…
- How to Analyze Photosynthesis in Plants: Methods and Tools
- Forest & Plant Canopy Analysis – Tools…
- The Forest Canopy: Structure, Roles & Measurement
- The Importance of Leaf Area Index (LAI) in…
- Root Respiration: Importance and Applications
- Stomatal Conductance: Functions, Measurement, and…
- Irrigating with Saline or Seawater
- Plant Respiration: Its Importance and Applications