April 7, 2022 at 9:49 pm | Updated April 20, 2022 at 1:48 pm | 12 min read
Precision agriculture is being branded as Agriculture 4 – the next revolution to make food production more profitable, sustainable, and environmentally friendly. Even though its adoption is uneven, the use of precision agriculture has been increasing consistently for the last several years. This article will review precision agriculture technologies, their adoption, and how precision agriculture policy is changing as we move toward 2023.
What is Precision Agriculture?
The definition of precision agriculture varies. According to Roberson (2001), it is a comprehensive system to optimize agricultural production by leveraging crop information, technology, and management practices. To be effective, the new approach should start in the crop planning stages and go beyond the farm to include post-harvest processing.
It is the management of food production by using technologies to acquire and analyze data to support decision making. It can help reduce input use, improve yields and ROI, increase quality food, and reduce the environmental impact of agriculture.
Subscribe to the CID Bio-Science Weekly article series.
By submitting this form, you are consenting to receive marketing emails from: . You can revoke your consent to receive emails at any time by using the SafeUnsubscribe® link, found at the bottom of every email. Emails are serviced by Constant Contact
How precision agriculture is practiced will differ widely based on geographical locations, type of farming, and stage of farming. It is as applicable in greenhouse cultivation, vertical indoor farms, and animal rearing as it is for open crop farms.
Data collection
Precision agriculture is usually understood to be the acquisition of multispectral and hyperspectral data through remote imagery from satellites and drones, or it is the acquisition of environmental data and soil and crop conditions through sensors on the farms. Many CID Bio-Science tools are useful for measuring stress, growth, and biomass accumulation:
- Minirhizotrons are used to monitor root growth and diseases.
- Leaf spectrometers and canopy imagers are used to detect stress due to abiotic and biotic stresses.
- Infrared gas analyzers are used to track biomass accumulation and stress by estimating photosynthesis, stomatal conductance, and transpiration.
- Near-infrared spectroscopy-based quality meters are used to estimate harvest time.
Analyzing information
Sensors come with associated chemometrics to analyze data in the same tool. Often, these sensors are part of the Internet of Things (IoT) in smart farming and are connected to automatic or semi-automatic control of inputs.
Remote data is analyzed separately by precision agriculture specialists like software providers. Several startups offering analytic services analyze remote data using artificial intelligence (AI), machine learning, and computers to provide insights into crop performance, which growers can use to make decisions. For example, data and analyzed maps show the difference in crop germination, growth, potential stressors, and stress intensity. This helps growers use the variable rate application (VRA) principle when making decisions.
Decision making
Armed with real-time data, growers can confidently decide the need, timing, and amount of fertilizers, chemicals, and irrigation to apply to their crops. In precision agriculture, the application of inputs is based on actual crop requirements and site-specific, intra-field differences. Due to microvariations in soil structure, fertility, and infestations, precision farming’s data-driven insights are preferable to conventional farming’s reliance on general recommendations for a region or cultivar.
- Farmers can apply the required amounts of chemicals to only infested areas to contain pests and diseases.
- High-performing parts of fields receive less or no additional fertilizers, while poor patches get more.
- Farmers can identify areas of over or under-irrigation
- Farmers can use yield maps to determine harvest time, field sequence, and optimal speed of operation.
Usually, large farms use “smart” or advanced farm machinery to provide inputs using VRA with the help of prescription maps. However, the development of VRA applicators for small farms is not well developed as of yet.
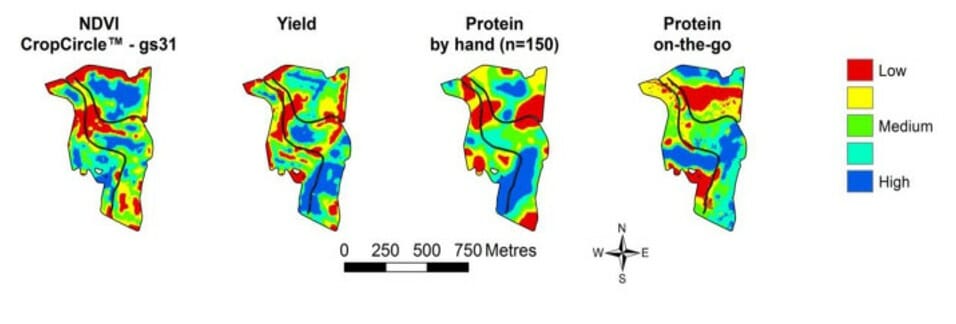
Result
By using the concept of precision agriculture, it is possible to provide the right amount of inputs where needed and optimize crop growth and health to improve yield and ROI. This approach also reduces the use of inputs and the associated expenditure. By limiting input use, there is a reduction in carbon emissions, agricultural waste, and pollution. Soil fertility is thus unaffected; however, the use of heavy machinery will still impact the soil.
The efficacy of precision farming in reducing inputs and improving yield varies according to crop, region, and technology used.
Post-Harvest Precision Agriculture
The application of data, technology, and decision-making that occurs post-harvest finds little mention during discussions of precision agriculture. The post-harvest stage is more critical in the case of horticultural crops than agricultural crops. After harvest, grains are cleaned and can be stored long without loss. Fresh produce—both vegetables and fruits—are perishable and require careful monitoring until they are sold.
For the last two decades, precision tools have been used for handling fresh produce. These can be handheld or mounted inline and online in large processing and sorting centers. They collect data that is then analyzed by integrated chemometrics to estimate various quality parameters like dry matter, sugar content, titrable acidity, and internal color.
Some standard post-harvest precision agriculture practices are as follows:
- Quality parameters are used as maturity indices to predict harvest time.
- Fresh produce is tested for sorting, grading, and packing according to its ripeness to optimize marketing decisions.
- Quality is monitored during storage, ripening, transport, and retailing.
- The atmosphere, containing ethylene, oxygen, and carbon dioxide, in which fresh produce is stored and ripened, is meticulously monitored and controlled by handheld or fixed tools.
- Grains are checked for toxins and pest infestations.
- Packers and retailers monitor fresh produce packaged in MAP packages.
- Fruit processing for juices, jams, winemaking, brewing, and more rely on internal quality estimation.
Result
As a result of this close monitoring, growers can cut losses at several critical stages. Waiting until the fruits are mature or ripe enhances quality and taste to meet consumers’ preferences and reduce the chances of rejection. Proper grading and sorting also prevent loss by removing damaged fruit and preserving products from spoiling due to ethylene formation.
Similarly, animal husbandry also uses precision tools (portable, online, and inline) to estimate the internal quality of fat, proteins, carbohydrates, and moisture after culling. Each species, subspecies, and even part of an animal have a different composition. These differences help food producers detect the origin of their raw materials, adulteration, processing method, and overall quality.
The dairy industry also uses tools for estimating the quality of feed, which reduces fodder use and carbon emissions. Also, handheld, online, and inline precision tools assess milk quality and are used for processing the milk into cheese.
These technologies, which use the same logic of information, analysis of internal quality, and decision making, should find a more prominent place in precision agriculture discussions. Moreover, they have been in use for two decades, yet there is not much information about their use patterns around the globe. In comparison, the adoption of standard precision farming technology is better recorded.
Precision Agriculture Adoption
Starting in the 1980s, the USA is the largest and earliest adopter of precision agriculture. Currently, 15-40% of large farms in the US use some form of precision technology, such as variable-rate technology or guidance systems. The small farms in the USA that make up 85% of the total farms do not use any precision technology.
Canadians and 20% of Australians, also with big farms, are the next largest adopters of the system. Western European countries began adoption a decade after the USA, and 68% of their small farms also use the technology.
- Currently, the UK is the largest adopter; Scotland with 85% is leading in the UK, followed by 43% of Irish farmers
- In Denmark, 80% of wheat and barley farmers and 30-40% of oilseed growers use the technology.
- In Germany, 30% of farmers use precision agriculture technology; in Hungary, it is only 9.9%
In the global south, countries like Argentina, Brazil, Turkey, and South Africa have started adopting this technology for farm management in the last decade. Currently, adoption in African and Asian countries remains relatively low due to the prevalence of small to medium-sized, single-owner farms.
Precision agriculture technologies are used to the greatest extent for wheat. Although rice is also a significant cereal produced globally, there are far lower recorded instances of the use of precision agriculture to grow paddy in China, India, and Indonesia.
Despite its benefits, the adoption of precision agriculture is uneven. Some of the reasons for this are discussed below.
Hurdles to Adoption of Precision Agriculture
Common hurdles to the adoption of precision agriculture globally are as follows:
- A lack of knowledge about the possibilities and benefits of this new approach and how farmers can apply these technologies still exists. While many farmers may have heard of the technique, it can be seen as being difficult to understand.
- Integrated platforms covering all aspects of the process, and people to help in predictive advice can be challenging to find. While training in standard practices is well established, people still have difficulty gaining access to services or training in precision agriculture in many areas of the world.
- Good broadband access is necessary for digital farming, but even developed countries like the USA do not have broadband in all rural areas, making it impossible to use certain web-based technologies.
- The initial financial investment can be high even for large farmers to make. Often, the costs of large-scale, specialized machinery or equipment needed to retrofit old machines can be considerable. At a time when the agricultural income from farms is decreasing, it can be difficult for farmers to invest in new and unknown technology.
In regions where small and medium-sized farms are the rule, adoption rates are even lower, as much of precision farming is, at present, geared towards large farms. Though, options are available that can significantly assist smaller farms in the form of small, handheld produce quality meters and plant stress monitoring devices. Moreover, small-plot farmers who can scout their entire farm easily do not need many large-scale precision farming services, which large farms do. Thus, most of the benefit in these small-plot-dominated regions comes from small-scale precision agriculture practices preharvest and post-harvest, like zonal soil management or portable quality estimation.
So, how have governments helped in promoting precision agriculture? To find out, we look to the agriculture policy of the leading adopters.
What is Agriculture Policy?
Each country has an agriculture policy to guide domestic agricultural production to ensure a secure food supply, food quality, and price stability for its people. These policies can encompass crop choices, inputs, technical and financial support, employment, price-fixing modes, land use and environment protection regulations, and more. In addition, economic development, international agency requirements, local conditions, and import-export concerns all play a part in shaping the policy.
Most of the primary adopters acknowledge the practice of precision agriculture in their country and regularly fund research on the topic at universities.
However, governments have done little to promote precision agriculture, and it has not been given significant importance in the past by most agriculture policy – though this trend is slowly changing. Government extension services by agents, officers, or specialists promoting precision agriculture are also lacking.
USA’s Precision Agriculture Policy
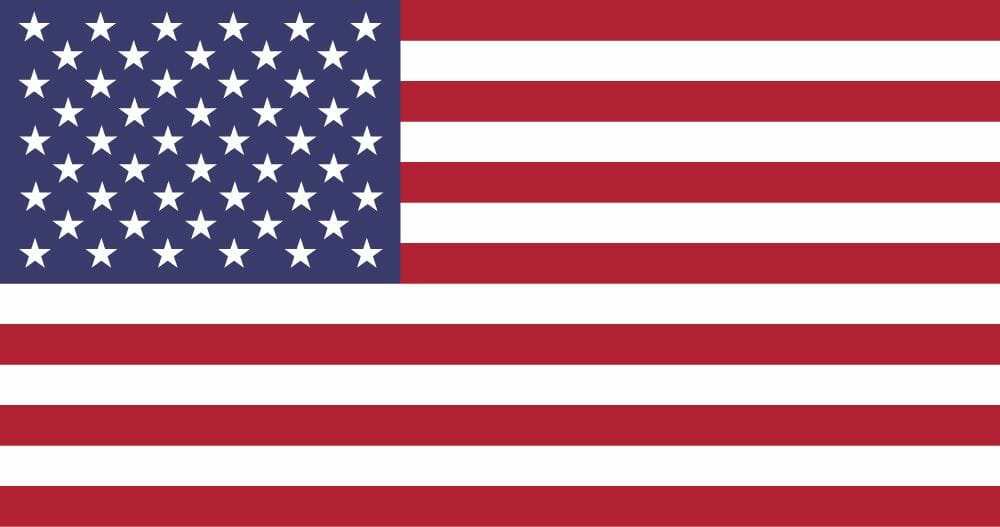
A study published in 2022 found that broadband internet access was lacking in rural areas of the USA. While information technology and “smart,” GPS-guided farming machinery require internet access, it was reported that only 26% of farmers had high-speed internet in rural areas. Recently, the US government has recognized the importance of correcting this situation to accelerate precision agriculture adoption. There is also renewed emphasis on resource management, climate-smart technologies, and sustainability.
To this end, the US government passed the Bipartisan Infrastructure Bill in August 2021, which aims to provide universal broadband access. The government hopes that from 2022, this measure will help the spread of precision farming to areas that so far have poor internet. However, many fear that the loss of emphasis on rural connectivity may adversely affect precision agriculture adoption.
At present, precision agriculture is not at the forefront of US agriculture policy. Precision agriculture specialists have advocated that the US government take the following policy steps in the future:
- Provide programs and resources to train farmers
- Ensure data privacy and security
- Connect precision agriculture with sustainability targets-social welfare, environmental protection, biodiversity conservation, etc.
- Educate and train extension services in the adoption of precision agriculture so that they can help in its promotion.
In the US, universities are the primary promoters for research, outreach, and development, often in cooperation with the private sector. Growers’ and input producers’ cooperatives have also been instrumental in introducing and spreading the technology. They often have agronomists and other precision agriculture specialists who can train and provide consultancy to growers in decision making. About 60% of input retailers provide precision agriculture services to their growers.
European Union’s Precision Agriculture Policy
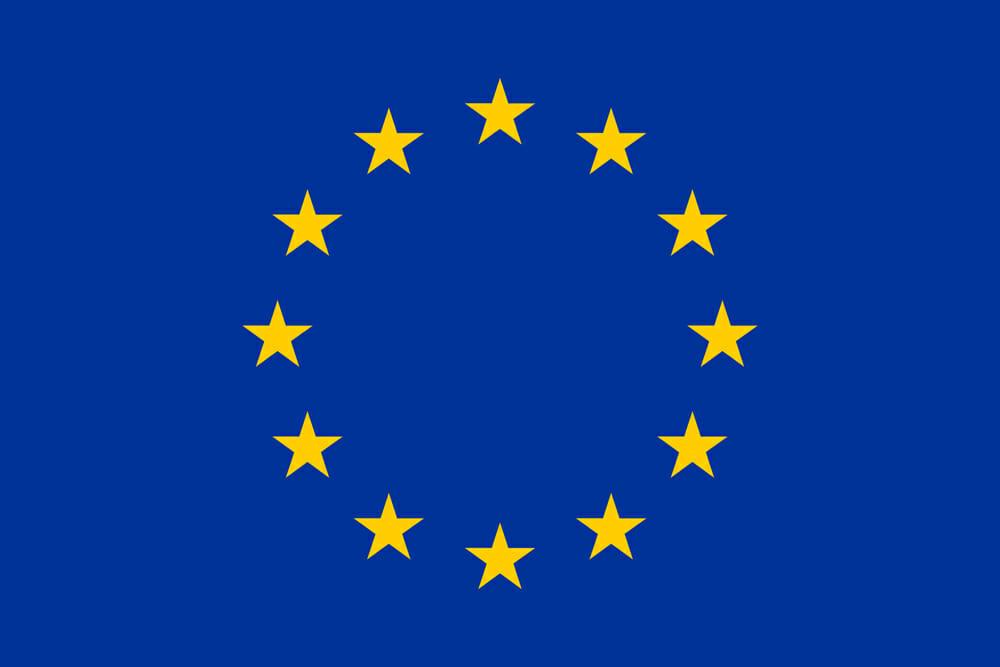
In contrast to the USA, where profit is the central driver of precision agriculture adoption, in the European Union (EU), concerns are more closely connected to the environment and productivity.
The government in the Netherlands has sponsored and promoted precision agriculture as one of the ways to improve their food production. The results are apparent in its rise in production.
The EU formulates the Common Agricultural Policy (CAP) for the bloc of 27 countries, which each nation then implements. The EU is just beginning to consider the various ideas of precision farming and plans to include it in its CAP to improve sustainable agriculture. Under the CAP strategies for 2023-2027, which are still being developed, precision agriculture is expected to be brought to the forefront in many ways. For example, investments are being explored in farm advisory training services and financial aid through eco-schemes for growers.
Precision agriculture specialists recommend the following to improve precision agriculture adoption in the EU:
- Identify the regions and typology of farms suitable for the technology
- Conduct pilot studies to formulate and evaluate programs
- Develop tools like precision farming calculators customized for different farming systems and include environmental benefits to aid growers
Australia’s Precision Agriculture Policy
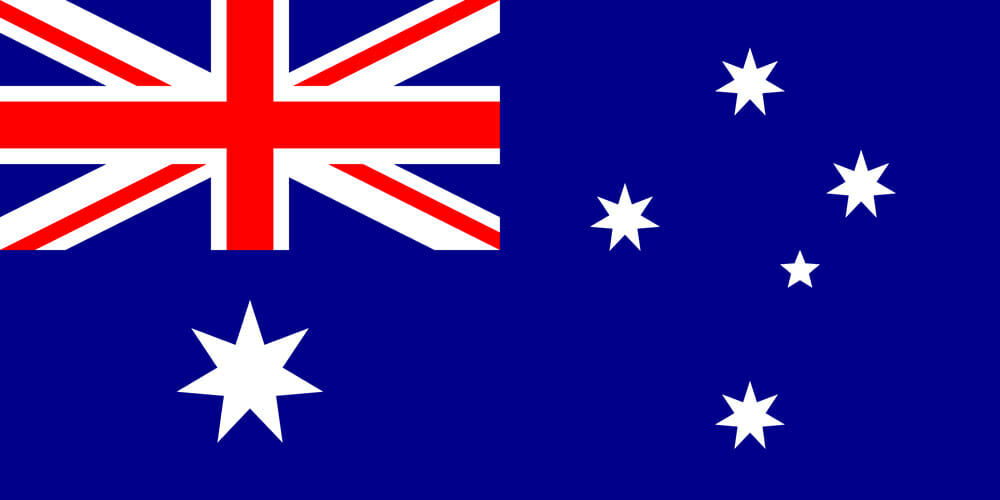
Australia is a major adopter of smart farming. In the agriculture policy announced in 2018, the Australian government has set aside over $27 million to promote digital technologies in agriculture to improve productivity and sustainability. It wanted Australia to become a world leader in adopting digital technologies.
By encouraging the use of technology in agriculture, the government hoped to attract the young generation to take up agriculture, stay in rural regions, and build the economy there. In 2018, it had announced the following measures to promote precision agriculture in Victoria:
- Provide internet connectivity to all rural areas.
- Train and educate the farmers on the use and benefits of digital farming.
- Set up agreed protocols for data sharing and privacy protection.
- Encourage integrated platforms that offer all related digital agriculture services in one place to make it easy for farmers to use.
- Start a $12 million pilot farm to test smart technologies for sheep, dairy, horticulture, and broadacre crops and share results with research groups and the private sector.
- Set up a regulatory structure to track innovations.
The policy has changed a little since then. In 2021, the Australian government started eight Innovation Hubs to promote research in digital technologies with $66 million and has given a further $114 million in 2022 to strengthen them. The hubs will connect researchers, investors, technology developers, and producers to improve innovation and development. In addition, shopfronts in forty locations will make the technology and locally relevant information widely and readily available to farmers.
With these measures, the Australian precision agriculture policy seems to be ahead of the other countries.
The Need for Precision Agriculture
Current agricultural practices will not be enough to feed an increasing population. No more arable land can be used, and the returns from existing farms are decreasing, mainly due to soil destruction. Heavy machinery destroys soil structure, and excessive chemicals are wiping out soil biodiversity. Also of concern is the erosion of topsoil and deforestation due to standard methods.
Add in the vagaries caused by climate change, and the future food supply begins to look very insecure.
Moreover, conventional farming methods have left many farmers debt-ridden due to high costs of inputs and low returns.
The excessive inputs that intensive agriculture relies on are also causing major environmental problems: pollution and climate change. Most fertilizers are lost as they seep into the ground and runoff during rainfall. The nutrient pollution caused by nitrogen and phosphorus causes eutrophication resulting in vast end zones in seas and impacting local water bodies.
Around 31% of the greenhouse gas comes from agriculture: 21% of CO2, 78% of nitrous oxide, and 53% of methane. Carbon emissions arise from fossil fuels used to manufacture fertilizers and pesticides and drive farm machinery. The unused nitrogenous fertilizers and manure are converted by bacteria to nitrous oxide, while methane is mainly produced by ruminant digestion in cattle and rice fields.
Food loss and food waste together contribute to 8-10% of total carbon emissions. Food loss occurs from farm to retail due to untimely harvest, improper packaging, sorting, transport, and storage.
Precision agriculture can reduce some of these negative impacts of intensive farming, mainly by reducing and optimizing input use.
Precision Agriculture Cannot Solve All Issues
Some people fear that the benefits of increased productivity and environmental protection are not enough to guarantee the high initial expenditure in precision agriculture. The input use has been reduced only by single-digit figures, whereas the promise is far more significant. Also, there are large gaps in the development of the approach. For example, it is not possible to measure soil phosphorus, so the information on soil fertility is not complete. Then there is the problem of control of data. Since large agrochemicals, inputs, GMOs, and agricultural machinery are investing in digital agriculture, there are fears that they will not allow the growers to get the full and potential benefits of the system. Will government agriculture policies be able to fix these issues? Moreover, other practices will also have to be changed to make agriculture sustainable.
—
Vijayalaxmi Kinhal
Science Writer, CID Bio-Science
Ph.D. Ecology and Environmental Science, B.Sc Agriculture
Sources
Arsenault, C. ( 2014). Only 60 Years of Farming Left If Soil Degradation Continues. Retrieved from https://www.scientificamerican.com/article/only-60-years-of-farming-left-if-soil-degradation-continues/
Australian Government. (n.d). Supporting agricultural innovation. Retrieved from https://www.awe.gov.au/sites/default/files/documents/factsheet-supporting-agricultural-innovation.pdf
Agriculture Victoria. (n.d.). Digital Agriculture Strategy. Retrieved from https://agriculture.vic.gov.au/__data/assets/pdf_file/0006/567051/Digital-agriculture-strategy-2018.pdf
Beste, A. (2021, Feb 7). Precision Farming – or “The Emperor’s New Clothes”? Retrieved from https://www.arc2020.eu/precision-farming-or-the-emperors-new-clothes/
Daniel Hellerstein, Dennis Vilorio, and Marc Ribaudo (editors). Agricultural Resources
and Environmental Indicators, 2019. EIB-208, U.S. Department of Agriculture, Economic
Research Service, May 2019
FAO. ( 2021). FAOSTAT ANALYTICAL BRIEF 31. The share of agri-food systems in total
greenhouse gas emissions Global, regional, and country trends 1990–2019. Retrieved from https://www.fao.org/3/cb7514en/cb7514en.pdf
Lee, C.-L.; Strong, R.; Dooley, K.E. Analyzing Precision Agriculture Adoption across the
Globe: A Systematic Review of Scholarship from 1999–2020. Sustainability 2021, 13, 10295. https://doi.org/10.3390/su131810295
Lencucha, R., Pal, N.E., Appau, A. et al. ( 2020). Government policy and agricultural production: a scoping review to inform research and policy on healthy agricultural commodities. Global Health, 16, 11. https://doi.org/10.1186/s12992-020-0542-2
Lynch, J., Cain, M., Frame, D., and Pierrehumbert, R. (2021) Agriculture’s Contribution to Climate Change and Role in Mitigation Is Distinct From Predominantly Fossil CO2-Emitting Sectors. Front. Sustain. Food Syst. 4:518039. DOI: 10.3389/fsufs.2020.518039
Mulla and Khosla. (n.d.). Historical Evolution and Recent Advances in Precision Farming. Retrieved from https://www.ispag.org/files/Mulla%20and%20Khosla%202015.pdf
Roberson, G.T. (2001). Precision Agriculture Technology for Horticultural Crop Production.
Vegetable Production & Marketing News, 11(3). Retrieved from https://aggie-horticulture.tamu.edu/extension/newsletters/vpmnews/mar01/art2mar.html
Sanders, C.E., Gibson, K.E., & Lamm, A.J. (2022). Rural Broadband and Precision Agriculture: A Frame Analysis of United States Federal Policy Outreach under the Biden Administration. Sustainability, 14, 460. https://doi.org/10.3390/su14010460
Schimmelpfennig, David. Farm Profits and Adoption of Precision Agriculture,
ERR-217, U.S. Department of Agriculture, Economic Research Service,
October 2016. www.ers.usda.gov/publications/err-economic-research-report/err217
UNFCC. (2020, Sept, 29). Fighting Food Waste Means Fighting Climate Change. Retrieved fromhttps://unfccc.int/news/fighting-food-waste-means-fighting-climate-change
Vermeulen, S. J., Campbell, B. M., & Ingram, J. S. (2012). Climate change and food systems. Annual review of environment and resources, 37, 195-222.
Wetzels, H. (2021, Nov 18). CAP beyond the EU | Precision agriculture: for whom precisely? Retrieved from https://eu.boell.org/en/2021/11/18/cap-beyond-eu-precision-agriculture-whom-precisely
Related Products
Most Popular Articles
- Transpiration in Plants: Its Importance and Applications
- Leaf Area – How & Why Measuring Leaf Area…
- How to Analyze Photosynthesis in Plants: Methods and Tools
- The Forest Canopy: Structure, Roles & Measurement
- Forest & Plant Canopy Analysis – Tools…
- Root Respiration: Importance and Applications
- Stomatal Conductance: Functions, Measurement, and…
- Plant Respiration: Its Importance and Applications
- The Importance of Leaf Area Index (LAI) in…
- Irrigating with Saline or Seawater