May 9, 2022 at 5:45 pm | Updated May 11, 2022 at 7:31 pm | 11 min read
Improving water use efficiency, the ratio of yield to water supplied can boost food production for a growing population without additional use of limited water resources. Water use efficiency for food production can be improved at the farm or plant level. On farms, this can be achieved through optimized management. At the plant level, improvements in water use efficiency can depend on manipulation of physiological and morphological features. In all cases, scientists need precise measurements in real-time, ideally taken in field conditions, to suggest new farming practices or develop new cultivars. In this article, we will discuss five critical approaches.
1. Optimize Leaf Area
Having more leaf area increases photosynthetic rates and biomass production. However, large leaves also result in higher rates of transpiration. This is not a problem when there is enough irrigation or rainfall, but plants experiencing water deficit or drought stress have varying WUE responses.
For this reason, crop physiologists measure water use efficiency (WUE) as yield per unit transpiration.
Subscribe to the CID Bio-Science Weekly article series.
By submitting this form, you are consenting to receive marketing emails from: . You can revoke your consent to receive emails at any time by using the SafeUnsubscribe® link, found at the bottom of every email. Emails are serviced by Constant Contact
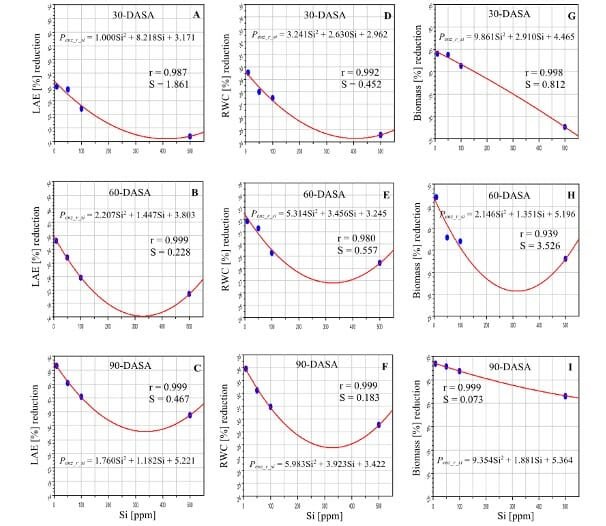
One plant response can be to reduce leaf area expansion to control transpiration, but that adversely affects biomass accumulation or yield. In addition, water deficit in plants can impact photosynthesis by injuring organelles like chloroplasts and mitochondria, and reducing the efficiency of photosystem II and chlorophyll production. Increased biosynthesis of reactive oxygen species (ROS) further damages the plant tissues.
An international group of agricultural scientists, Verma, Song, Tian, Singh, Verma, Rajput, Singh, Sharma, Singh, Malviya, and Li, provided silicon to sugarcane (Saccharum officinarum L). Silicon can be beneficial in mitigating the water stress effects in plants, as it accumulates in epidermal and vascular tissues in monocots and improves water uptake.
Sugarcane is a crop with one of the highest water and nutrient demands. Moreover, efforts are underway to introduce this important cash crop to water deficit regions, so finding a way to help the crop cope with water stress is crucial.
Sugarcane propagated from cuttings was grown in pots under similar conditions during the initial growth phase until 90 days. Then, the experiment started by withholding irrigation to maintain water stress at 45-50% soil moisture capacity and applying three levels of silicon. Calcium metasilicate, the silicon source, was added to irrigation water to supply 50, 100, and 500 ppm of Si L–1 for 40 days, and then three times each month. The control had 85-90% soil moisture capacity and no silicon. Scientists observed the plant performance after 30, 60, and 90 days of silicon addition.
The scientists used CID Bio-Science’s CI-203 Handheld Laser Leaf Area Meter to measure leaf area expansion (LAE), 30, 60, and 90 days after silicon treatment. The leaves were then used to estimate dry weight.
The scientists tested other leaf parameters, such as leaf relative water content, chlorophyll content, chlorophyll index (SPAD units), photosynthesis, transpiration rate, stomatal conductance, Chl a fluorescence (Fv/Fm), and antioxidant activity.
As expected, drought caused a 51% loss in leaf area expansion after 90 days. Scientists could increase leaf water content by 29-34% by adding silicon. The loss in leaf area expansion fell by 15-46% as silicon levels increased. Sugarcane that was given 500 ppm Si L–1 suffered the least. As a result, there were gains in biomass accumulation, see Figure 1.
The increase in leaf water content also increased stomatal conductance. As a result, there was also more transpiration and photosynthesis activity. For 500ppm of silicon, in 30, 60, and 90 days, respectively, scientists observed a reduction in photosynthesis loss by 25-29, 20-26, and 17-20%; a reduction in the loss of stomatal conductance activity by 28-38, 24-34, and 19-28%; and a reduction in transpiration loss by 49-52, 48-51, and 43-47%.
High silicon content in leaves improved the biosynthesis of chlorophyll and limited its decomposition. As a result, the photosynthesis rate improved. Moreover, silicon was able to regulate ROS effects by activating antioxidative enzymes.
The experiment showed that crops grown at just 50% soil moisture content could withstand drought stress and recover when they got 500 ppm silicon.
2. Improve the Rhizosphere and Root Dynamics
Biostimulants, which improve the rhizosphere, can boost fruit tree performance during drought stress.
The rhizosphere is the soil zone around the roots, which attracts microbes that use root exudates for their metabolism. Many of these microbes are beneficial as they are involved in nutrient cycling.
Webber experimented to see if biostimulants could improve water uptake by increasing root volume and distribution of roots in a two-year-long experiment on newly planted ‘Granny Smith/MM109’ apples.
Young apple transplants have most of their roots removed when transferred from the nursery to the field. The compromised root system, transplant shock, and apple replant disease (ARD) caused by soil pathogens can produce stunted trees. Fumigants (1, 3-D-Chloropicrin) applied to control the ARD also kill beneficial microflora, creating more stress.
The scientist tested the ability of biostimulants to reduce transplant shock and improve vegetative growth. In a second experiment, the scientist tested the effect of biostimulants in rehabilitating soil microbial communities and enhancing root growth dynamics.
Young Granny Smith apple trees were treated with six biostimulants Mycorrhiza, L-AA, three formulations of Trichoderma-based products (Trich 1 & Trich 2), plant extract, and compost. Webber compared these six treatments with untreated control. The young transplants were watered by hand twice a week.
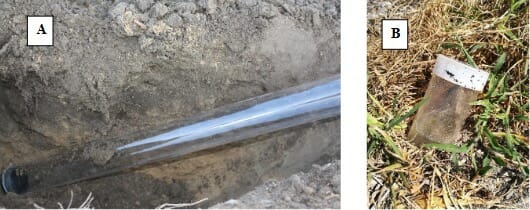
Three replicates from each treatment and the control were used for taking root scans with a minirhizotron root scanner, the CI-600 In-Situ Root Imager, manufactured by CID Bio-Science Inc. A clear, 100 cm long tube was installed at a 45° angle to the soil surface, about 10 cm from the trunk, to make measurements each month, see Figure 2. Four images were taken at soil depths of 45 – 60 cm, 30 – 45 cm, 15 – 30 cm, and 0 – 15 cm. In the first year, all treatments were scanned. But in the second year, only control, compost, and plant extract treatments were observed, and the images were analyzed by Root Snap Image Analysis Software (CI-690, CID Bio-Science Inc.). Root numbers, length, diameter, volume, and dead roots were counted, see Figure 3. This non-destructive method was also compared with a destructive root analysis.
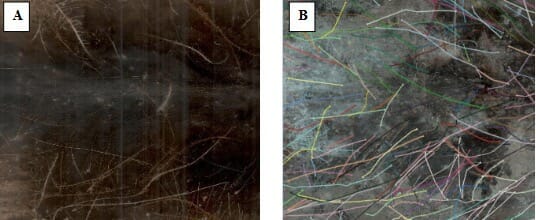
The biostimulants helped root dynamics, but the efficacy differed among treatments. Initially, Trich 1 and 2 treatments had the highest, lowest total root numbers and root length, and the Mycorrhiza the least, followed by control, compost, plant extract, and L-AA. But seasonal trends were opposite, with the highest increases seen in Mycorrhiza treatment over the season and least in Trich 1 and 2 treatments.
Webber also estimated leaf nutrients, stem diameters, shoot growth, total soluble sugars, photosynthetic capacity, stomatal conductance, and stem water potential to test the effect on vegetative parameters.
However, the biostimulants did not affect vegetative growth during the two years; and there were no differences between treatments. There was a drought in the second year. Still, the anticipated mitigating effect on water use efficiency, through increased root growth, did not materialize, not even in the Mycorrhiza treatment, which produced the best root volume improvements. One of the reasons could be that two years is too short for the results to manifest in perennial trees. Moreover, the biostimulants were applied only once. The scientist recommends further experiments with more frequent biostimulant applications to see above-ground effects.
3. Deeper Root Systems
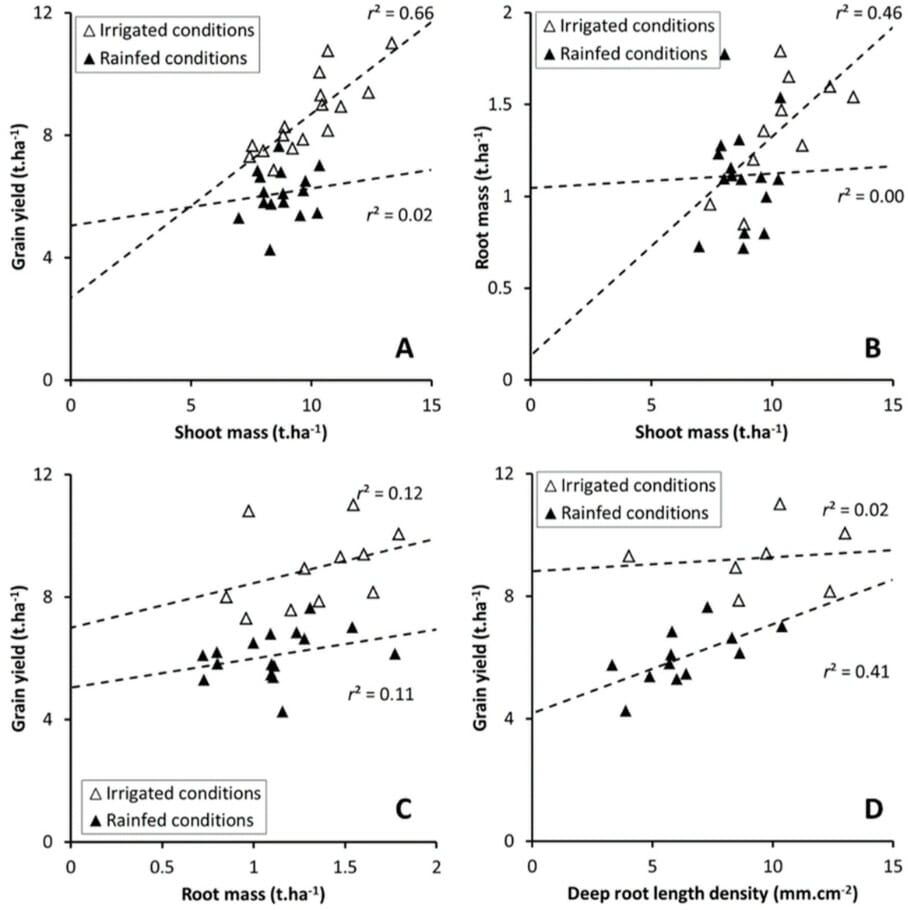
When breeding crops, scientists need phenotyping tools to evaluate cultivars.
Crops have different root architectures, influencing their ability to capture water and resist drought. Top rooted plants can exploit water better in wetter soils, but deeper roots are more helpful in dry soils. Lateral root growth and branching angles are other root parameters that influence resistance and drought tolerance.
Field experiments that combine soil type, nutrient deficiency, and environmental exposure provide a more realistic picture of soil-root interactions. However, field studies are limited because soil coring, shovelomics, and root quantification methods are destructive, cumbersome, and time-consuming.
Hence, plant scientists, Postic, Beauchêne, Gouache, and Doussan, used scanner-based minirhizotrons as field phenotyping tools for root study. They tested four varieties of wheat, Apache, Hystar, Nogal, and Miradoux (durum wheat), for four combinations of irrigation and nitrogen (I + N+), (I+ N-), (I- N+), and (I- N-).
To get root scans, they installed root tubes to a depth of 122 cm after wheat emergence. Then they collected scans using the CID Bio-Sciences’ CI-600 In-situ Root Scanner at tillering, stem elongation, flag leaf ligule, and halfway through anthesis. The Smartroot software processed the images to measure root length, diameter, volume, and surface densities. Soil augers sampling provided root biomass estimations.
Shoot dry mass and nitrogen content at different growth stages were also estimated.
Water deficiency had more impact than nutrient deficiency. Wheat grown in rainfed and irrigated conditions showed varying root development and yields, but the yield was always related to root surface density at anthesis.
In rainfed and nutrient-deficient conditions, wheat varieties developing deeper roots produced greater yield. The shoot biomass did not influence the yield, see Figure 4.
Whereas in irrigated plots, the yield was proportion to shoot biomass. Their roots, which were concentrated in the topsoil, did not affect yield.
The variety Apache had the deepest roots in rainfed and nutrient-deficient soils. It had greater drought tolerance as it could increase its capacity for water capture compared to Miradoux, which has a shallower root system. The performance of the other two varieties was intermediate and more mixed.
The scientists concluded that data on root parameters and growth patterns is essential for crop breeding programs to understand plant response to drought, as vegetative parameters are not always relevant to yield.
4. Leaf Area Index
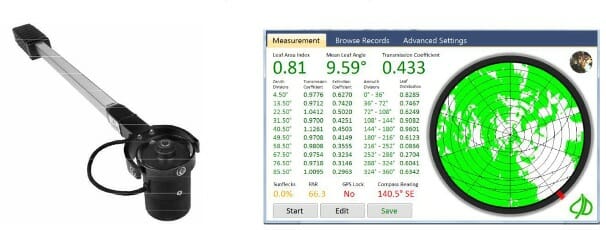
Leaf area index (LAI), a canopy parameter, can provide more information than the leaf area alone. LAI is the one-sided leaf area per unit area. It includes information on the total surface of the plant or trees available for gas exchanges, rain interception, and other vegetation links to the environment. LAI is useful for scaling estimates of photosynthesis and evapotranspiration.
The importance of rain interception in providing water to rainfed orchards or even irrigated fields in arid regions cannot be overestimated. In their leafless conditions, the woody structures are estimated in LAI in deciduous species and play a vital role in determining plant water balance.
Different tree species will have varying LAI and are linked to fruit tree yield. There are several direct and indirect methods to measure LAI.
Horticulturists Patil, Biradar, Bhagawathi, and Hejjegar describe the estimation of LAI in fruit trees like guava using the CI-110 Digital Plant Canopy Imager produced by CID Bio-Science Inc. The imager has a fisheye lens that takes hemispherical images from beneath the canopy, as shown in Figure 5. They chose this method as it is non-destructive, faster, and automated, allowing more spatial sample collection.
There is little information about the LAI of horticultural crops compared to annuals. So more research is needed in this area.
5. Optimizing Irrigation
Water use efficiency by crops can be improved by field management and choice of irrigation. Horticultural crops, like grapes, need irrigation to balance yield and fruit quality in arid regions. Using localized surface drip systems can lead to salinity, soil hypoxia, and water loss through leaching.
Novel subsurface micro-irrigation systems can reduce evapotranspiration, restrict water to weeds, improve crop WUE, and regulate grapevine growth.
Direct root-zone irrigation (DRZ) is one such method that is growing popular and can improve yield by 9-12% compared to surface drip (SD).
However, there isn’t enough information on the physiological performance of grapevines under this new system. Grapes need deep roots for support, but the possibility of DRZ changing rooting patterns remains unchecked.
Ma, Jacoby, and Sanguinet, crop and plant scientists, conducted a two-year study in a commercial
Cabernet Sauvignon orchard in a semi-arid region, to compare DRZ and SD under three watering regimes- high (0.75–0.80 ETc), moderate (0.60–0.65 ETc), and low (0.45–0.50 ETc). Irrigation was applied from bud-break to post-harvest.
They compared specific leaf area, leaf gas exchanges, stem water potential, nitrogen and total carbon content in leaves and shoots, and root distribution in a 0-160 cm soil profile. CID Bio-Science’s CI-340 Handheld Photosynthesis System can be used to measure all the gas exchanges, providing vital information on photosynthetic activity.
Minirhizotron tubes of 1.8 m depth were installed 30 cm away from the vines in the soil. Eight images were taken at different depths using the CI-600 In Situ Root Imager, produced by CID Bio-Science Inc., at three stages each year- fruit set, veraison, and harvest. The scientists analyzed the root number and root length density.
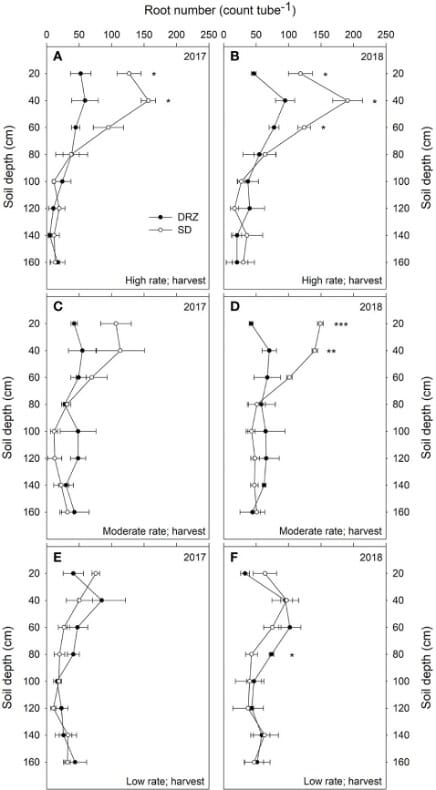
Decrease in irrigation, and the DRZ method improved WUE in grapevines. Water provided at the subsurface decreases water loss through evaporation, leaving more for the grapevines.
There were no differences in the two systems at low rates of irrigation, see Figure 6. At moderate and high irrigation rates, DRZ results in 50-60% lower total root number and 30-40% lower root length in the upper 60 cm of soil. However, in deeper soils (60-160 cm), DRZ produces a higher root number and length density. This is accompanied by increased leaf area and decreased total carbon to nitrogen in leaves.
Thus, the stem water potential is higher in DRZ irrigation, and higher stomatal conductance, or more open stomata, result in higher photosynthesis and transpiration.
The difference in the two irrigation systems was most apparent in the dry summer months and dry years, where DRZ irrigated plants showed higher yield. The development of deeper roots it produces helps in water stress amelioration and adaptation to the arid climate.
More Methods
The five listed examples are a selection of the methods and approaches that exist to measure WUE. It is impossible to cover all of them in a review. Several other management practices and irrigation methods are currently being investigated as field-level interventions. Similarly, genetic and other physiological processes exist to breed varieties with WUE strategies suited for specific geographies and climatic conditions. Armed with modern technology, precise data, and analytical approaches, scientists are helping to improve WUE in our systems globally.
—
Vijayalaxmi Kinhal
Science Writer, CID Bio-Science
Ph.D. Ecology and Environmental Science, B.Sc Agriculture
Sources
Ma, X., Jacoby, P. W., & Sanguinet, K. A. (2020). Improving net photosynthetic rate and rooting depth of grapevines through a novel irrigation strategy in a semi-arid climate. Frontiers in Plant Science, 11. https://doi.org/10.3389/fpls.2020.575303
Mbava N., Mutema M., Zengeni R., et al. (2020). Factors affecting crop water use efficiency: a worldwide meta-analysis. Agricultural Water Management, 228, p. art. 105878 [11 p.]. ISSN 0378-3774.
Postic, F., Beauchêne, K., Gouache, D., & Doussan, C. (2019). Scanner-based Minirhizotrons help to highlight relations between deep roots and yield in various wheat cultivars under combined water and nitrogen deficit conditions. Agronomy, 9(6), 297. https://doi.org/10.3390/agronomy9060297
Verma, K. K., Song, X.-P., Tian, D.-D., Singh, M., Verma, C. L., Rajput, V. D., Singh, R. K., Sharma, A., Singh, P., Malviya, M. K., & Li, Y.-R. (2021). Investigation of defensive role of silicon during drought stress induced by irrigation capacity in sugarcane: Physiological and biochemical characteristics. ACS Omega, 6(30), 19811–19821. https://doi.org/10.1021/acsomega.1c02519
Webber, M. (2021). The effect of biostimulants on the performance of newly planted apple trees in fumigated soil. [Master thesis]. Stellenbosch University. Retrieved from
Related Products
Most Popular Articles
- Transpiration in Plants: Its Importance and Applications
- Leaf Area – How & Why Measuring Leaf Area…
- How to Analyze Photosynthesis in Plants: Methods and Tools
- Forest & Plant Canopy Analysis – Tools…
- The Forest Canopy: Structure, Roles & Measurement
- The Importance of Leaf Area Index (LAI) in…
- Root Respiration: Importance and Applications
- Stomatal Conductance: Functions, Measurement, and…
- Irrigating with Saline or Seawater
- Plant Respiration: Its Importance and Applications